This article was originally written by John T. Streicher.
The intent of this document is to explain some of the issues related to powering a typical AC drive from a generator source. Some of the information given is theoretical and some data shown comes from actual installations. Since each system configuration and performance requirements are unique it is impossible to give hard and fast numbers that will guarantee satisfactory operation. It is the hope of the author that this document will give others a better understanding of the drive / generator system and help those responsible for the installation to make it a successful one.
Application
There are two basic ways a generator can be used as a power source, stand-by (back up) or permanent (dedicated). In a system such as a storm sewer pumping application, the generator may be used for stand by (back up) power. On an oil rig, generators may be the only power source. Whatever the application, generators are increasingly being used to supply power for AC drives. A good understanding of the drive, the generator and how each affects the other is essential to assure proper operation of the final system.
Drive Basics
Lets start by looking at the basic designs and power requirements of an AC drive. Most drives use either 6 SCR’s (Silicon Controlled Rectifier) or 6 diodes to create a full wave three phase bridge rectifier. For a three phase system, each phase creates 2 pulses resulting in what is called a 6 pulse rectified converter. The converter section is often refered to as the “front end”. The front end converts the 3 phase AC line to a DC voltage which is then filtered with large bulk capacitors (Bus caps) and in some cases an inductor (link choke). This DC power is then used by the inverter stage of the drive to send a synthesized sine wave of the required voltage and frequency to control the speed and direction of the AC motor.
Figure 1: Diode Bridge / Inverter
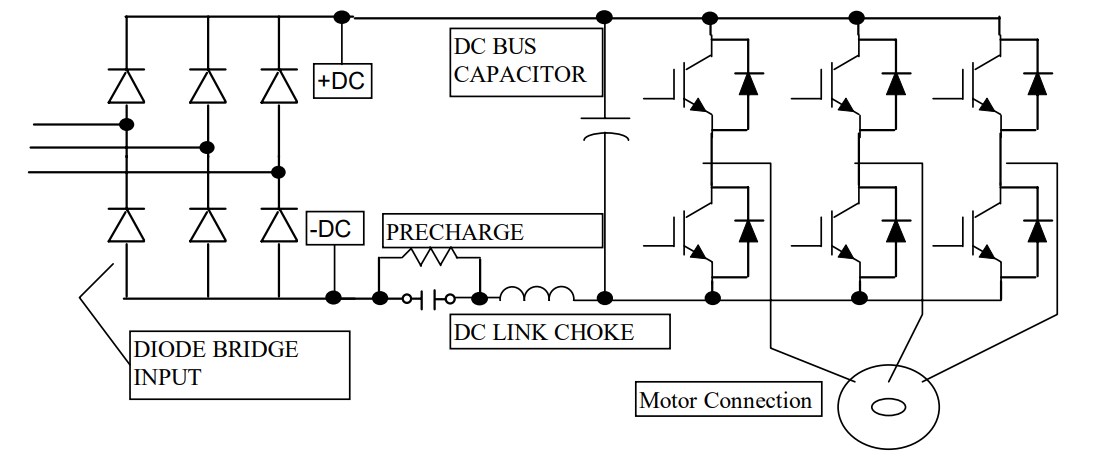
A diode bridge input configuration is normally used where the input current is about 50 amps or less. In the case of the diode front end, input frequency is not an issue and wide variations in input frequency may be tolerated. Each diode simply conducts during the part of the cycle when it is forward biased. Inrush to the capacitor bank upon power up is taken care of usually by a resistor “precharge” circuit in parallel with ether a relay or a solid state switch.
Figure 2: SCR Bridge / Inverter
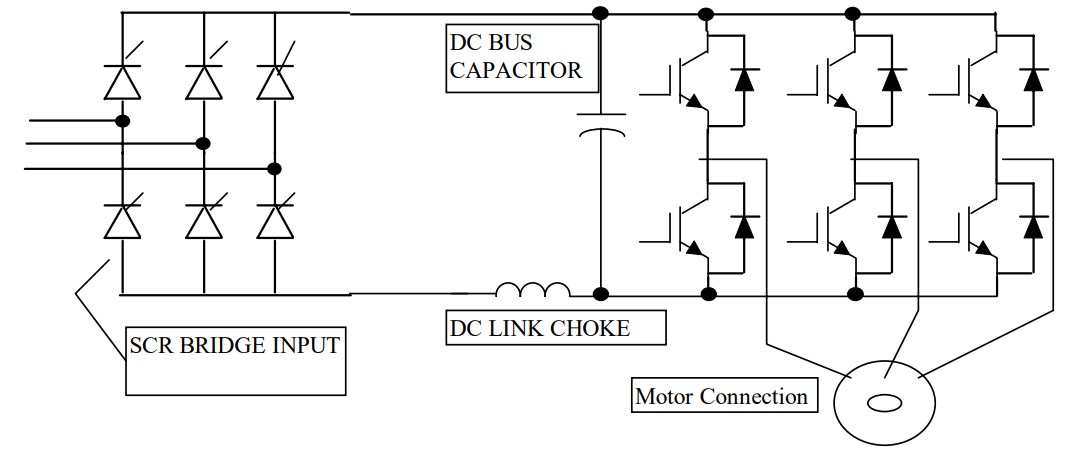
In drives where input current is 50 amps or more, an SCR input bridge is more common. Refer to figure 2. With the SCR bridge, inrush may be controlled by the input gate firing angle. Since an SCR will begin conducting during the positive half of the cycle only after a gate pulse is applied, controlling the timing or firing angle (sometimes called “Alpha”) of the gates on the SCR bridge allows the DC bus voltage to be ramped up. This limits inrush or “precharges” the drive. This technique requires that the SCR control circuit be synchronized to the power line.
It must also be mentioned that an SCR has a higher leakage current if the power connections are reverse biased and a gate signal is present. To minimize the leakage and make the SCR bridge more efficient, the gate signals are normally not present during the time the SCR is reverse biased. This requires a tracking circuit that follows the input line frequency. For this reason, generator output frequency regulation may be more important on a drive with an SCR front end. Considering that line starting a motor can draw 6 to 10 times running current levels, and soft starting the motor with a drive keeps both motor and line current below 100% of the motor rated current, it’s easy to see why one would want to use a drive. A drive will control motor starting current. However, the drive’s input current from the line is very different from motor line current when the motor is directly across the line. The motor running directly from the line will have a nice sine wave shape but a relatively poor power factor of between 0.2 and 0.85 depending on load. With the drive installed, the input to the drive will have good power factor (about .95). However, because it is a rectifier, the current is no longer in the shape of a sine wave. The rectifier at the front end of an AC drive pulls current mostly at the peak of the sine wave since the only time current can flow is when the AC line peak voltage is higher than the DC bus capacitor voltage. This type of load is said to be “ Nonlinear”.
Generator Basics
Now lets look at the generator. A generator consists of two basic parts. The engine or prime mover and the alternator or generator which converts the mechanical power of the engine to electric power. Both parts have control systems. The alternator or generator has a voltage regulator and the engine has a speed governor or regulator. Alternators are typically employed above 50KW. The engine may be powered by Gasoline, Diesel, Natural gas or perhaps Steam. In any case it is the frequency (mechanical speed) and voltage output that ultimately need to be controlled.
Generators As A Source Of Power
Naturally most generator manufactures want to give their machines the highest KW rating possible. Usually they allow a power factor of about 0.8 and do not make allowances for a nonlinear load. Furthermore it is very typical of a generator to have a 20% source impedance as opposed to a 3% to 5% impedance for an equivalent size power transformer on the utility line. Since the voltage drop in a circuit is proportional to the impedance of a circuit, the generator output voltage drops most during the portion of the cycle where the current is highest. In the case of most nonlinear / rectified loads this is at the peak of the sine wave. Thus it is the high impedance source and the nonlinear load that combine to distort the output voltage wave-form of the generator. The result is an output where the RMS voltage may be correct but the peak to peak voltage may be 70% or less of an undistorted sine wave output. If one compares the true sine wave and the distorted wave-form with an equivalent RMS value, the area under the curve will be the same for a given time period. However, any nonlinear rectified load on the high impedance source will have a much lower DC voltage level. This is because the DC bus is now limited to the lower peak of the distorted wave-form.
Figure 3: Computer simulated wave-forms of input current and voltage for a lightly loaded generator
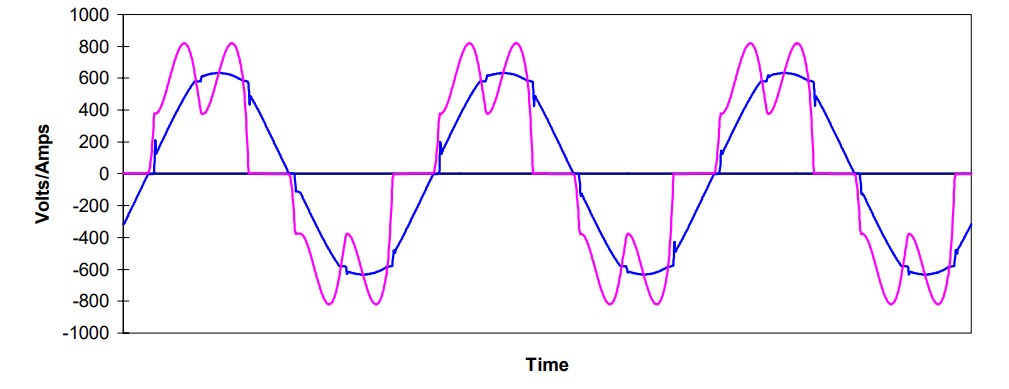
Figure 4: Computer simulated wave-forms of input current and voltage for a heavily loaded generator
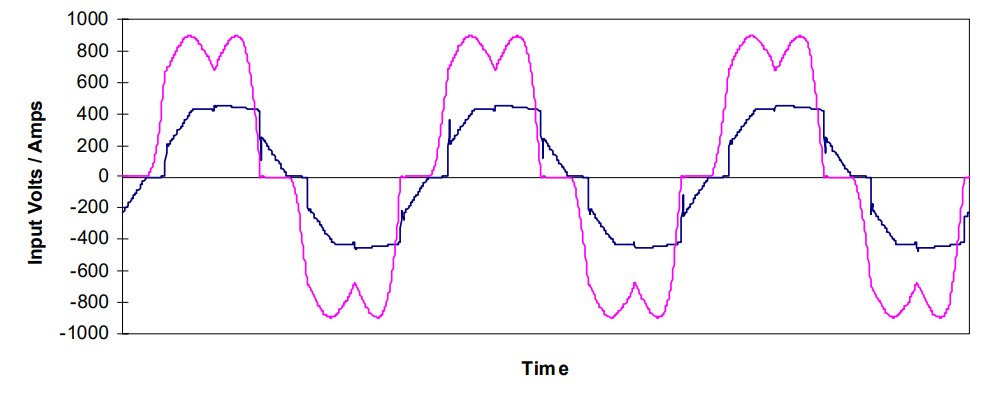
Notice how the voltage output from the generator becomes distorted under load. Peak voltage drops from over 600 volts to just over 400 volts. If this were a real generator system, the regulator would call for more voltage. The goal of the regulator in most cases is to satisfy the RMS voltage desired. The output would be increased until the area under the curve is such that the RMS voltage is correct. This does little to control the “flat topping”. For a rectified input, the DC bus voltage will reflect this change. The DC bus voltage will drop significantly under load.
Figure 5: Computer simulated current and voltage of a loaded, 3% impedance transformer on the utility line.
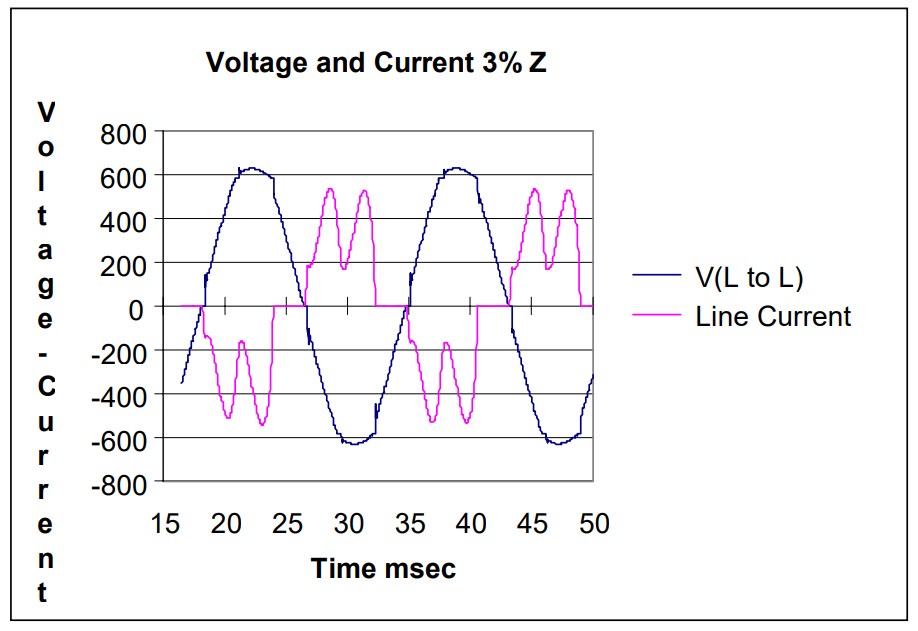
Notice that the peak voltage of the transformer on the utility line does not drop significantly. A rectified load feed from this source would not incur an appreciable DC voltage dip due to loading. Historically, generators have been used on linear loads such as lights and motors running across the line. These types of loads pull current equally over the line cycle and do not distort the output voltage. These types of loads may also be more tolerant to poor voltage and frequency regulation. In most cases a distorted output voltage wave shape or slight variation in frequency would not significantly change the speed of a motor or intensity of the lights powered from it.
However, in the case of the AC drive we have the “precharge” issue to deal with. A distorted line voltage results in a lower DC bus voltage since the filter capacitors are charging to the new, lower peak value of the distorted voltage wave-form. Furthermore, abrupt variations in line frequency can confuse the AC line tracking circuit and cause an SCR bridge to fire the gates at the wrong time. This too can result in a lower DC bus voltage. The drive may see this as a line dip. With the understanding that “what goes down, must come up”, the drive may try to protect itself from a current inrush by going into “precharge” mode. While in precharge mode, the drive will cease output to the motor since full or even partial power can not be supplied through a precharge resistor or an SCR front end that is phased back.
One can see how this could cause an interruption in whatever process the drive motor combination is being used on. In some cases this interruption is not a problem and the end user will live with the poor performance. Other processes are not as forgiving. In many cases however, the drive gets stuck in a cycle. Load, and associated distortion, causes a precharge condition. While the drive is in “precharge”, the generator sees a reduced load and the voltage distortion goes away. The DC bus in the drive then charges to the higher peak of the undistorted wave-form, comes out of “precharge” and runs the motor. Eventually the accelerating motor will want more power Distortion at the generator output voltage will build and trigger another precharge cycle. If left alone this condition may eventually clear itself. This is because precharge is triggered by the difference between the instintanious DC buss level and a time weighted average. Multiple attempts at loading the generator will bring down the time weighted average DC level thus reducing the sensitivity to precharge cycling.
Application Tips
Let’s look at what can be done to improve performance where a high impedance source and / or poor voltage and frequency regulation may cause problems. For large systems where many generators and drives are used, try to spread out and evenly distribute the loads such that drives and other nonlinear loads do not exceed 20% of the total generator capacity. If more than one drive must be run from a given generator, a phase shifting technique can be employed using a 12 pulse transformer. This is a transformer with one primary and two secondary windings usually configured as a Delta (primary), Delta/ Y (secondary). This method more evenly distributes the current demand by making the nonlinear portion of the load on the generator look like a 12 pulse system even though 6 pulse drives are being used. This technique can be expanded to three or more drives using the required number of transformer secondary windings; each with a phase shift of (60 deg/n) where n is the number of drives or nonlinear loads.
A more costly but effective solution is to use a harmonic trap filter. These filters essentially force current draw from the AC line or generator at 60 Hertz. The filter stores energy during part of the line cycle and delivers it to the drive or nonlinear load during the part of the cycle requiring the large peak currents.
Another method of dealing with nonlinear loads is to oversize the Alternator portion of the generator, effectively lowering the source impedance of the generator. Increasing the engine size, in general, will not help improve voltage distortion.
In some cases special generator firmware may be installed in the drive that will allow a programmable precharge level. This will permit the drive to run with the low DC bus caused by the distorted generator output. This feature takes advantage of the fact that the generator, with it’s high source impedance, does not have the ability to damage the drive input with a current inrush. The level of the precharge should be set no lower than needed to run the drive on the generator. Furthermore, an input is typically provided on the generator firmware to deactivate this feature while the drive is run from utility power. If the feature is not deactivated while on the utility power the low source impedance of the utility line could cause serious dammage to the drive.
If a drive with an SCR input is used, we must also be concerned with frequency regulation since the bridge controller is usually synchronized to the power line. In this case a larger engine will help since more rotating inertia and available torque should improve speed regulation. Adding a large flywheel to increase only the rotating inertia will help by limiting the rate of change of the speed of the motor and thus limit the rate of change of the line frequency. It is the rate of change of frequency that can cause problems. A slower rate of change will allow the SCR bridge controller a greater chance to follow the changing line frequency. Sudden changes in generator load (both increasing and decreasing) should be avoided since this will affect the output frequency. Transfer load to and from the generator in stages if possible.
In some cases a dynamic interaction can occur causing an oscillation condition between the drive front end and the generator. This condition can occur on a running system or at start up. It usually occurs with a sudden change in load on the generator resulting in a change in line frequency or output voltage or both. This causes the drive to go into it’s precharge mode where output to the motor is cut off. The power demand at the input of the drive is now small as the drive waits for the line to stabilize. The now reduced load allows the generator to recover. Once stable, the drive may try to resume output to the motor at the last commanded frequency resulting in a sudden load change to the generator which causes a change in line frequency and / or voltage. This cycle can continue unless steps are taken to create a way out of this loop. The way out is to set up the drive such that, after a precharge takes place, the drive and motor must ramp up to speed, avoiding the shock load condition.
Remember, even when running from Utility Power, the source of the power is ultimately a generator. The difference is that the utility power line has very low impedance and the utility generator is very large relative to the drive load. The utility also has much greater load diversity. This load diversity causes cancellation of harmonics through phase shifting and summation of the variations in load current in the many branch circuits on the power grid.
Things To Avoid
Do not use a line reactor between the drive and generator as this only adds impedance. Do not assume that if the Kilowatt rating of a generator exceeds the total load Kilowatt rating that the generator is large enough. Avoid programming the drive to do a “Flying Start” after a line loss or power loss ride through. Keep speed reference signals as noise free as possible. Try to avoid shock loading the generator as this can cause oscillations that the generator voltage regulator and speed governor may have trouble recovering from. Noisy speed reference signals can cause a shock load or create an oscillating condition that a generator may have trouble recovering from.
Things To Improve Performance
Set generator voltage as high as the equipment being run will allow. This will minimize voltage distortion by reducing current demand for a given load point. If using a backup generator, run Utility Line Voltage slightly low. This will minimize the % change in the DC bus voltage when transferring from one source to another. Limit nonlinear loads to no more than 20% of the generator capacity.
Use ”Phase Shifting” techniques to reduce total harmonics. Oversize the Alternator portion of the generator by at least 3X the required load. If the drive has an SCR bridge controller, make sure the tracking circuit has a “Phase Locked Loop” to allow fast tracking response to line frequency changes.
Use custom generator firmware if available. Make sure to set the adjustable pre-charge level properly (no lower than needed. Also if the drive is alternating between a generator and the utility, use the input provided to signal the drive as to the power source.
Example
Shown are two actual plots taken from an installation where AC drives running pumps are powered from a generator source. The top wave-form in each case is the input voltage and the lower wave-form is input current. The top plot shows little voltage distortion under light load. The bottom plot shows a great deal more voltage distortion under heavy load of about 75% of the generator capacity. This installation was marginal and was only able to work after adjustments to the generator output voltage, filtering to remove noise on the 4-20 ma input speed signal and programming the drive to ramp from 0HZ after a power transfer from utility line to the stand-by generator.
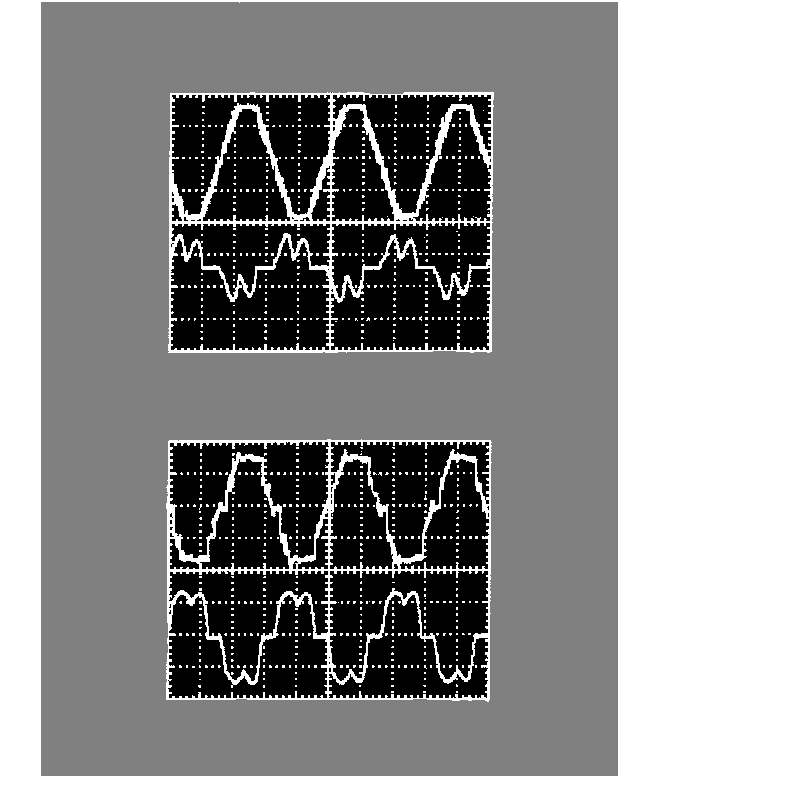